Novel High-Throughput Micro/Nanofluidic Technology
Author: Omar Heikal
Learning Objectives
- Describe the synergy between the cell retention system (microfluidic device) and product quality monitoring system (nanofluidic system)
- List the limitations and advancements, individual components, method for perfusion culture, and effect of electric field strength on separation performance of the micro/nanofluidic system
- Evaluate the technology’s contribution to long-term and efficient continuous biomanufacturing
Graphical Abstract
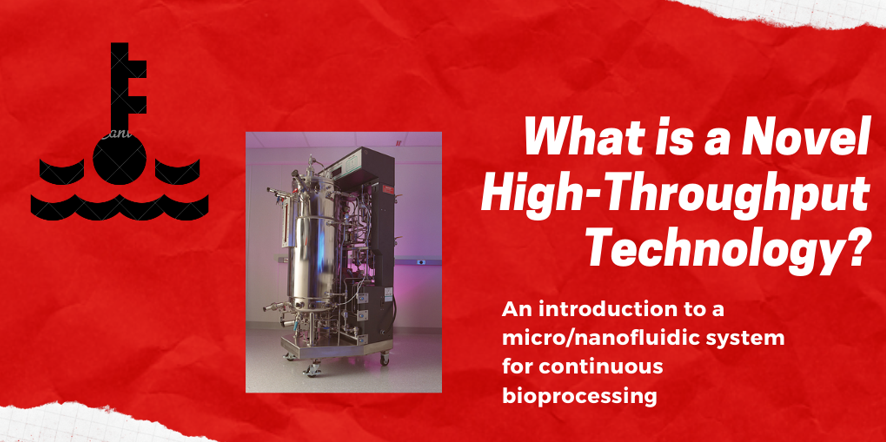
Introduction/Background
In efforts to improve product quality and biomanufacturing efficiency, a novel micro/nanofluidic system was created. This innovative high-throughput technology targeted the continuous biomanufacturing trend that has transformed modern medicine. The integrated system overcame the limitations of current membrane-based microfiltration and quality monitoring technologies— filter clogging, low product recovery, manual sample preparation, and offline analysis [2]. Whilst the in-use perfusion bioreactors continuously produced the culture supernatant containing monoclonal immunoglobin antibodies (IgG1), novel high-throughput microfluidic cell separation was put to the test using a cell retention device with clog-free cell retention [3]. High cell concentrations of greater than 20 million cells/mL of suspended IgG1-producing Chinese hamster ovary (CHO) cells were separated by membrane-less microfiltration via size-dependent hydrodynamic forces. This initial step underscored a lab-scale cell retention device that was reliable and prototypical for mass production involving rapid perfusion processes [3]. The microfluidic spiral device was then used to continuously remove small and nonviable suspended CHO cells from bioreactors. The test cell concentration capacity and volumetric throughput were increased to optimize typical bioreactor limits [2]. Lastly, a novel nanofluidic filter device was employed to continuously monitor protein product size and quantity for several days to a week. Successfully revolutionizing conventional and offline analytical technologies for protein purity monitoring, this on-site monitoring system served its purpose during perfusion culture production [1].
Using standard soft lithography, a 3D modeling tool (Rhinoceros, McNeel North America, USA) was ran to engineer the microfluidic channels of the microfluidic device. The model incorporated a single spiral channel of eight loops and 300 μm-wide inner outlet, and four spiral channels in parallel with six loops and enlarged inner outlet 520 μm in width. These channels were fabricated with aluminum metal using micromachining [3]. On a hotplate, a polydimethylsiloxane (PDMS) elastomer solution was poured into these molds at 150 oC for 15 minutes. This created the assembled PDMS slab bonded to a flat glass slide. Afterwards, silicone tubing (Masterflex, Cole-Parmer, USA) for transferring fluid was inserted into the inlets and outlets of the microchannel. The drilled, glass side of the device was then placed on top of the microchannel and clamped [3].
The nanofluidic device was manufactured using a multiple standard microelectromechanical systems (MEMS) method. Photolithography and dry etching were initially done on a silicone substrate with a 5X reduced stepper (NSR2005i9, Nikon Precision Inc.) and reactive-ion etching (RIE), respectively. Wet etching with potassium hydroxide (KOH) was then used to make the access holes for loading samples and applying electric field [4]. For electrical insulation, an additional thermal oxide layer (SiO2) was stacked between the silicon substrate and buffer solution. And a fusion bonding method fixed the silicon and Pyrex glass together to prevent the nanochannel from collapse. Finally, a dicing saw was used to cut the bonded substrates with precision [4]. This proof-of-concept prototype proved to be of merit when the at-line monitoring system for purity assessment was demonstrated. The nanofilter array was connected with an on-line sample-preparation system to analyze the supernatants with IgG1. The pressurized, treated sample was then directly loaded onto the array via electro-osmosis and/or electrophoresis [1]. And microchannels were placed at each end to account for leaks. Upon analysis, the nanofluidic device seemed to perform better than expected. It not only monitored the heavy chain (AbH), light chain (AbL), and impurities (aggregates and host-cell proteins) of the supernatant, but it also continuously and instantaneously monitored changes of biomolecule property (i.e. different IgG1 concentrations) [1].
This novel high-throughput technology did not only meet or exceed the safety and assurance standards that we all know, but it also exceeded many conventional state-of-the-art technologies. Its analysis system measured aggregation, folding, and binding activity automatically with precision [1]. Nonetheless, the on-line sample preparation system did continue to have a sample-to-assay wait time of five hours which can be more significant if managed. In modern bioreactors, notable results appear after tens of hours. On-line sample preparation, however, can be optimized with the use of a non-covalent dye or label-free detection method like ultraviolet fluorescence. The capillary system of the microfluidic device can also be switched with a sample preparation workflow of significantly less dead volume [1].
Truly a one-of-a-kind, next generation, and biomanufacturing platform, this micro/nanofluidic system should be recognized for its contribution to society. It will simplify and expedite product release and drug administration to the patient which will benefit many lives. People will have increased access to medicines that they need to be treated for acute disorders that would have been potentially life-threatening. The high-throughput aspect of this technology has been refined, essentially producing sufficient output that will not only reduce manufacturing cost but will also improve product quality. It is important to publicize technological innovations such as this one because it protects any shared intellectual property and it gives others a chance to use or enhance the technology.
Audio Recroding
References
[1] Ko, S. H., Chandra, D., Ouyang, W., Kwon, T., Karande, P., & Han, J. Nanofluidic device for continuous multiparameter quality assurance of biologics. (2017). Nature Nanotechnology, 12(8), 804–812. https://doi.org/10.1038/NNANO.2017.74 [2] Kwon, T., Han, J. Advanced Micro/nanofluidic System for Continuous Bioprocessing. Research Laboratory of Electronics at MIT (2020). https://www.rle.mit.edu/micronano/bioprocessing/ [3] Kwon, T., Prentice, H., Oliveira, J. De, Madziva, N., Warkiani, M. E., Hamel, J.-F. P., & Han, J. Microfluidic Cell Retention Device for Perfusion of Mammalian Suspension Culture. (2017). Scientific Reports, 7, 6703. https://doi.org/10.1038/s41598-017-06949-8 [4] Kwon, T., Sung H.K., Jean-François P.H., and Jongyoon H. Continuous Online Protein Quality Monitoring during Perfusion Culture Production Using an Integrated Micro/Nanofluidic System. Analytical Chemistry (2020). 92 (7), 5267-5275. DOI: 10.1021/acs.analchem.9b05835 [5] (2008). Retrieved from https://images.app.goo.gl/zqT4N77ZFfmMdWac9
Questions
- What is this? This novel high-throughput technology provides an alternative solution for long-term reliable and efficient continuous biomanufacturing. This research introduces technologies that are used for high-throughput cell separation and nanofluidic product quality monitoring. It involves a cell retention system (microfluidic device) that produces perfusion culture, provides a long-term, clog-free operation, and dead cell/cluster removal. The other integrated part is a product quality monitoring system (nanofluidic device) that features continuous, online monitoring that is fully automated offering rapid purity/bioactivity check [2].
- Why is it important? This advanced micro/nanofluidic system is an important development for modern medical application and advancing treatments for many ailments. It overcomes the limitations of conventional microfiltration and protein quality monitoring during biomanufacturing. The device’s parameters have been optimized in regard to cell retention capacity, biocompatibility, scalability, and long-term reliability [3].
- List learning objectives.
Describe the synergy between the cell retention system (microfluidic device) and product quality monitoring system (nanofluidic system)
List the limitations and advancements, individual components, method for perfusion culture, and effect of electric field strength on separation performance of the micro/nanofluidic system
Evaluate the technology’s contribution to long-term and efficient continuous biomanufacturing - Reflect This research makes me think of the aims of this course to educate and critically think about high-throughput discovery science. As a student and fellow scholar, I enjoyed exploring a novel high-throughput technology that has created a dent in the field of biotechnology. I am now aware of the depth of continuous biomanufacturing as it relates to myself. I believe that this assay will have tremendous potential in the pharmaceutical industry and change the future in a better direction. But this technological advancement among many others will be superseded as many others before. This does not necessarily indicate that this micro/nanofluidic system is faulty. It merely reflects a sign of the times.
- How does this approach, technology, or concept relate to social and environmental justice…? Although this innovative technology may not be relevant in matters of religion or culture, I think it does relate to social and environmental justice in some regard. An introduction of a robust and commercially applicable biotechnology such as this micro/nanofluidic system would have significant impacts on healthcare and society. By reducing production costs and duration of medicine, more people will have increased access to a better standard of care and consequent quality of living. Socioeconomic statuses will be altered and potentially leveled. Moreover, the bioreactors that is used in conjunction with this device involves chemical processes that include organisms and/or biologically active substances which are derived from the environment. Although bioreactors effectively control environmental conditions, it disregards environmental justice to some extent when it mimics natural living processes and uses cells or tissues in culture.